Diamant sintetic: Diferență între versiuni
(Nicio diferență)
|
Versiunea de la 2 martie 2013 00:04
Unul sau mai mulți editori lucrează în prezent la această pagină sau secțiune. Pentru a evita conflictele de editare și alte confuzii creatorul solicită ca, pentru o perioadă scurtă de timp, această pagină să nu fie editată inutil sau nominalizată pentru ștergere în această etapă incipientă de dezvoltare, chiar dacă există unele lacune de conținut. Dacă observați că nu au mai avut loc modificări de 10 zile puteți șterge această etichetă. |
Diamantul sintetic (cunoscut și ca diamant de laborator, diamant crescut în laborator sau diamant cultivat) este un diamant produs cu ajutorul unui procedeu artificial, spre deosebire de diamantele naturale, care sunt create printr-un proces geologic în natură. Mai sunt cunoscute denumirile de diamant HPHT și de diamant CVD, date după metodele de producție folosite. [1].
Deși adesea s-a folosit termenul de sintetic, acesta a fost considerat cumva problematic. În Statele Unite, Federal Trade Commission a indicat faptul că termenii de diamant crescut în laborator sau creat de [un anumit] producător "ar transmite mai clar natura pietrei", pentru că anumiți consumatori asociază termenul de sintetic cu ideea că produsul este o imitație – întrucât diamantele fabricate artificial sunt efectiv diamante. [2]
Numeroase încercări pentru sintetizarea diamantului au fost făcute între anii 1879 și 1928; multe dintre aceste încercări au fost cu grijă analizate dar niciuna nu a fost confirmată. În anii 1940, au început cercetări sistematice pentru creșterea diamantelor prin procesele CVD și HPHT [1] în Statele Unite, Suedia și Uniunea Sovietică . Prima sinteză reproductibilă a fost raportată în jurul anului 1953, iar aceste două procedee încă domină producția de diamante sintetice. O a treia metodă, cunoascută ca detonare, și-a făcut apariția la sfârșitul anilor 1990. În acest proces, granule de diamant de dimensiunea câtorva nanometri sunt create prin detonarea unor explozivi conținători de carbon. Într-o a patra metodă, demonstrată în laborator, dar fără aplicații comerciale în prezent, grafitul este tratat cu ultrasunete de mare putere.
Proprietățile diamantului sintetic sunt în strânsă legătură cu detaliile procedeului de fabricație; totuși, unele diamante sintetice (formate prin HPHT sau CVD) au proprietățile ca duritatea, conductivitatea termică sau mobilitatea electronilor superioare chiar față de unele diamante naturale.
Diamantele sintetice sunt utilizate pe larg ca abrazivi, la tăierea și șlefuirea uneltelor și în radiatoarele electrice. Aplicațiile electronice ale diamantelor sintetice, printre care se numără comutatoarele de la centralele electrice, tranzistozii unipolari și LED-urile, sunt în dezvoltare. Detectoarele cu diamante sintetice de lumină ultravioletă (UV) sau de particule cu energie mare sunt folosite în domenul energiei înalte și sunt disponibile comercial. Datorită combinației unice dintre stabilitatea sa termică și chimică, expansiunii termale mici și transparenței optice mari, diamantul sintetic tinde să devină cel mai popular material folosit ca sticlă optică pentru laserele cu dioxid de carbon și girotroane.
Ambele diamante de tip CVD și HPHT pot fi tăiate ca geme și se pot produce culori variate: alb pur, galben, brun, albastru, verde și portocaliu. Aspectul gemelor sintetice de pe piață a adus neliniște în rândul afacerilor cu diamante, și ca rezultat aparate spectroscopice și tehnici speciale au fost dezvoltate pentru a se putea face diferența între diamantele sintetice și cele naturale.
Istoric

După descoperirea din anul 1797 că diamantul este carbon pur, au fost realizate multe încercări de a tranforma diferite varietăți ieftine de carbon în diamant. Primele succese au fost raportate de către James Ballantyne Hannay în 1879 [3] și Ferdinand Frédéric Henri Moissan în 1893. Metoda lor implica încălzirea mangalului până la 3500 °C cu fier în interiorul unui creuzet de carbon într-un cuptor. Întrucât Hannay a folosit o țeavă încălzită direct în flacără, Moissan a practicat metoda sa recent descoperită , cuptorul electric cu arc, în care un arc electric era lovit între tije de carbon în interiorul unor blocuri de calcar. [4] Fierul topit era apoi răcit rapid prin scufundare în apă. Contracția generată de răcirea fierului se presupune că a produs presiunea înaltă necesară pentru a transforma grafitul în diamant. Moissan și-a publicat rezultatele într-o serie de articole în anii 1890. [5]
Mulți alți savanți au încercat să refacă experimentul său. William Crookes pretindea că a reușit în 1909. [6] Otto Ruff susținea în 1917 că a produs diamante de până la 7 mm în diametru, [7] dar ulterior și-a retras afirmația. [8] În 1926, doctorul J.Willard Hershey de la McPherson College a refăcut experimentele lui Moissan și ale lui Ruff, [9][10] producând astfel un diamant sintetic; acest eșantion este expus la Muzeul McPherson din Kansas. [11] În ciuda celor pretinse de Moissan, Ruff și Hershey, alte experimente nu au fost capabile să reproducă sinteza lor. [12][13]
Cea mai definitivă încercare de replicare au fost făcute de Charles Algernon Parsons. Un savant proeminent și inginer cunoscut pentru inventarea variantei moderne a turbinei cu aburi, el și-a irosit aproximativ 40 de ani (1882–1922) și o mare parte a averii sale încercând să reproducă experimentele lui Moissan și ale lui Hannay, dar de asemenea și el și-a adaptat procese proprii. [14] Parsons era cunoscut pentru abordarea sa precisă și migăloasă și pentru păstrarea metodicii sale de înregistrare; toate eșantioanele sale au fost conservate pentru ulterioare analize de către un partid independent. [15] El a scris un număr de articole — unele dintre primele referitoare la diamantele HPHT—în care el a susținut că a reușit producerea a mici diamante. [16] Totuși, în 1928 el l-a autorizat pe doctorul C.H. Desch să publice un articol [17] în care și-a prezentat părerea că niciun diamant sintetic (incluzându-le chiar și pe ale lui Moissan și ale altora) nu au fost de fapt produse până atunci. El a sugerat că toate diamantele care au fost produse până atunci erau cel mai probabil eșantioane de spinel. [12]
Proiectul diamant GE

În 1941 a fost făcut un acord între companiile General Electric (GE), Norton și Carborundum pentru a dezvolta sinteza diamantelor. Acestea era în stare să încălzească carbonul la aproximativ 3000°C la o presiune de 3,5 gigapascali pentru cțteva secunde. Curând după aceea, al Doilea Război Mondial a întrerupt proiectul. El a fost reluat în 1951 la Laboratoarele Schenectady ale GE, iar un grup de diamante de înaltă presiune a fost format cu F.P. Bundy și H.M. Strong. Tracy Hall și mulți alții s-au alăturat proiectului la scurt timp după. [18]
The Schenectady group improved on the anvils designed by Percy Bridgman, who received a Nobel Prize for his work in 1946. Bundy and Strong made the first improvements, then more were made by Hall. The GE team used tungsten carbide anvils within a hydraulic press to squeeze the carbonaceous sample held in a catlinite container, the finished grit being squeezed out of the container into a gasket. The team recorded diamond synthesis on one occasion, but the experiment could not be reproduced because of uncertain synthesis conditions,[19] and the diamond was later shown to have been a natural diamond used as a seed.[20]
Hall achieved the first commercially successful synthesis of diamond on December 16, 1954, and this was announced on February 15, 1955. His breakthrough was using a "belt" press, which was capable of producing pressures above 10 GPa (1.500.000 psi) and temperatures above 2.000 °C (3.630 °F).[21] The press used a pyrophyllite container in which graphite was dissolved within molten nickel, cobalt or iron. Those metals acted as a "solvent-catalyst", which both dissolved carbon and accelerated its conversion into diamond. The largest diamond he produced was 0,15 mm (0,0059 in) across; it was too small and visually imperfect for jewelry, but usable in industrial abrasives. Hall's co-workers were able to replicate his work, and the discovery was published in the major journal Nature.[22][23] He was the first person to grow a synthetic diamond with a reproducible, verifiable and well-documented process. He left GE in 1955, and three years later developed a new apparatus for the synthesis of diamond—a tetrahedral press with four anvils—to avoid violating a U.S. Department of Commerce secrecy order on the GE patent applications.[20][24] Hall received the American Chemical Society Award for Creative Invention for his work in diamond synthesis.[25]
Later developments
An independent diamond synthesis was achieved on February 16, 1953 in Stockholm by the ASEA (Allmänna Svenska Elektriska Aktiebolaget), one of Sweden's major electrical manufacturing companies. Starting in 1949, ASEA employed a team of five scientists and engineers as part of a top-secret diamond-making project code-named QUINTUS. The team used a bulky split-sphere apparatus designed by Baltzar von Platen and Anders Kämpe.[18][26] Pressure was maintained within the device at an estimated 8.4 GPa for an hour. A few small diamonds were produced, but not of gem quality or size. The work was not reported until the 1980s.[27] During the 1980s, a new competitor emerged in Korea, a company named Iljin Diamond; it was followed by hundreds of Chinese enterprises. Iljin Diamond allegedly accomplished diamond synthesis in 1988 by misappropriating trade secrets from GE via a Korean former GE employee.[28][29]

Synthetic gem-quality diamond crystals were first produced in 1970 by GE, then reported in 1971. The first successes used a pyrophyllite tube seeded at each end with thin pieces of diamond. The graphite feed material was placed in the center and the metal solvent (nickel) between the graphite and the seeds. The container was heated and the pressure was raised to about 5.5 GPa. The crystals grow as they flow from the center to the ends of the tube, and extending the length of the process produces larger crystals. Initially a week-long growth process produced gem-quality stones of around 5 mm (1 carat or 0.2 g), and the process conditions had to be as stable as possible. The graphite feed was soon replaced by diamond grit because that allowed much better control of the shape of the final crystal.[23]
The first gem-quality stones were always yellow to brown in color because of contamination with nitrogen. Inclusions were common, especially "plate-like" ones from the nickel. Removing all nitrogen from the process by adding aluminium or titanium produced colorless "white" stones, and removing the nitrogen and adding boron produced blue ones.[30] Removing nitrogen also slowed the growth process and reduced the crystalline quality, so the process was normally run with nitrogen present.
Although the GE stones and natural diamonds were chemically identical, their physical properties were not the same. The colorless stones produced strong fluorescence and phosphorescence under short-wavelength ultraviolet light, but were inert under long-wave UV. Among natural diamonds, only the rarer blue gems exhibit these properties. Unlike natural diamonds, all the GE stones showed strong yellow fluorescence under X-rays.[31] The De Beers Diamond Research Laboratory has grown stones of up to 25 carate (5,0 g) for research purposes. Stable HPHT conditions were kept for six weeks to grow high-quality diamonds of this size. For economic reasons, the growth of most synthetic diamonds is terminated when they reach a mass of 1 carat (200 mg) to 1,5 carate (300 mg).[32]
In the 1950s, research started in the Soviet Union and the US on the growth of diamond by pyrolysis of hydrocarbon gases at the relatively low temperature of 800 °C. This low-pressure process is known as chemical vapor deposition (CVD). William G. Eversole reportedly achieved vapor deposition of diamond over diamond substrate in 1953, but it was not reported until 1962.[33] Diamond film deposition was independently reproduced by Angus and coworkers in 1968[34] and by Deryagin and Fedoseev in 1970.[35] Whereas Eversole and Angus used large, expensive, single-crystal diamonds as substrates, Deryagin and Fedoseev succeeded in making diamond films on non-diamond materials (silicon and metals), which led to massive research on inexpensive diamond coatings in the 1980s.[36]
Manufacturing technologies
There are several methods used to produce synthetic diamond. The original method uses high pressure and high temperature (HPHT) and is still widely used because of its relatively low cost. The process involves large presses that can weigh hundreds of tons to produce a pressure of 5 GPa at 1500 °C. The second method, using chemical vapor deposition (CVD), creates a carbon plasma over a substrate onto which the carbon atoms deposit to form diamond. Other methods include explosive formation (forming detonation nanodiamonds) and sonication of graphite solutions.[37][38][39]
High pressure, high temperature
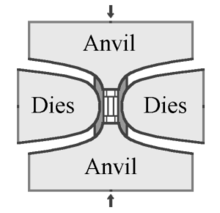
In the HPHT method, there are three main press designs used to supply the pressure and temperature necessary to produce synthetic diamond: the belt press, the cubic press and the split-sphere (BARS) press.
The original GE invention by Tracy Hall uses the belt press wherein the upper and lower anvils supply the pressure load to a cylindrical inner cell. This internal pressure is confined radially by a belt of pre-stressed steel bands. The anvils also serve as electrodes providing electrical current to the compressed cell. A variation of the belt press uses hydraulic pressure, rather than steel belts, to confine the internal pressure.[40] Belt presses are still used today, but they are built on a much larger scale than those of the original design.[41]
The second type of press design is the cubic press. A cubic press has six anvils which provide pressure simultaneously onto all faces of a cube-shaped volume.[42] The first multi-anvil press design was a tetrahedral press, using four anvils to converge upon a tetrahedron-shaped volume.[43] The cubic press was created shortly thereafter to increase the volume to which pressure could be applied. A cubic press is typically smaller than a belt press and can more rapidly achieve the pressure and temperature necessary to create synthetic diamond. However, cubic presses cannot be easily scaled up to larger volumes: the pressurized volume can be increased by using larger anvils, but this also increases the amount of force needed on the anvils to achieve the same pressure. An alternative is to decrease the surface area to volume ratio of the pressurized volume, by using more anvils to converge upon a higher-order platonic solid, such as a dodecahedron. However, such a press would be complex and difficult to manufacture.[42]

The BARS apparatus is the most compact, efficient, and economical of all the diamond-producing presses. In the center of a BARS device, there is a ceramic cylindrical "synthesis capsule" of about 2 cm3 in size. The cell is placed into a cube of pressure-transmitting material, such as pyrophyllite ceramics, which is pressed by inner anvils made from cemented carbide (e.g., tungsten carbide or VK10 hard alloy).[44] The outer octahedral cavity is pressed by 8 steel outer anvils. After mounting, the whole assembly is locked in a disc-type barrel with a diameter about 1 meter. The barrel is filled with oil, which pressurizes upon heating, and the oil pressure is transferred to the central cell. The synthesis capsule is heated up by a coaxial graphite heater and the temperature is measured with a thermocouple.[45]
Chemical vapor deposition
Chemical vapor deposition is a method by which diamond can be grown from a hydrocarbon gas mixture. Since the early 1980s, this method has been the subject of intensive worldwide research. Whereas the mass-production of high-quality diamond crystals make the HPHT process the more suitable choice for industrial applications, the flexibility and simplicity of CVD setups explain the popularity of CVD growth in laboratory research. The advantages of CVD diamond growth include the ability to grow diamond over large areas and on various substrates, and the fine control over the chemical impurities and thus properties of the diamond produced. Unlike HPHT, CVD process does not require high pressures, as the growth typically occurs at pressures under 27 kPa.[37][46]
The CVD growth involves substrate preparation, feeding varying amounts of gases into a chamber and energizing them. The substrate preparation includes choosing an appropriate material and its crystallographic orientation; cleaning it, often with a diamond powder to abrade a non-diamond substrate; and optimizing the substrate temperature (about 800 °C) during the growth through a series of test runs. The gases always include a carbon source, typically methane, and hydrogen with a typical ratio of 1:99. Hydrogen is essential because it selectively etches off non-diamond carbon. The gases are ionized into chemically active radicals in the growth chamber using microwave power, a hot filament, an arc discharge, a welding torch, a laser, an electron beam, or other means.
During the growth, the chamber materials are etched off by the plasma and can incorporate into the growing diamond. In particular, CVD diamond is often contaminated by silicon originating from the silica windows of the growth chamber or from the silicon substrate.[47] Therefore, silica windows are either avoided or moved away from the substrate. Boron-containing species in the chamber, even at very low trace levels, also make it unsuitable for the growth of pure diamond.[37][46][48]
Detonation of explosives

Diamond nanocrystals (5 nm in diameter) can be formed by detonating certain carbon-containing explosives in a metal chamber. These nanocrystals are called "detonation nanodiamond". During the explosion, the pressure and temperature in the chamber become high enough to convert the carbon of the explosives into diamond. Being immersed in water, the chamber cools rapidly after the explosion, suppressing conversion of newly produced diamond into more stable graphite.[49] In a variation of this technique, a metal tube filled with graphite powder is placed in the detonation chamber. The explosion heats and compresses the graphite to an extent sufficient for its conversion into diamond.[50] The product is always rich in graphite and other non-diamond carbon forms and requires prolonged boiling in hot nitric acid (about 1 day at 250 °C) to dissolve them.[38] The recovered nanodiamond powder is used primarily in polishing applications. It is mainly produced in China, Russia and Belarus and started reaching the market in bulk quantities by the early 2000s.[51]
Ultrasound cavitation
Micron-sized diamond crystals can be synthesized from a suspension of graphite in organic liquid at atmospheric pressure and room temperature using ultrasonic cavitation. The diamond yield is about 10% of the initial graphite weight. The estimated cost of diamond produced by this method is comparable to that of the HPHT method; the crystalline perfection of the product is significantly worse for the ultrasonic synthesis. This technique requires relatively simple equipment and procedures, but it has only been reported by two research groups, and has no industrial use la data de 2012[update]. Numerous process parameters, such as preparation of the initial graphite powder, the choice of ultrasonic power, synthesis time and the solvent, are not yet optimized, leaving a window for potential improvement of the efficiency and reduction of the cost of the ultrasonic synthesis.[39][52]
Properties
Traditionally, the absence of crystal flaws is considered to be the most important quality of a diamond. Purity and high crystalline perfection make diamonds transparent and clear, whereas its hardness, optical dispersion (luster) and chemical stability (combined with marketing), make it a popular gemstone. High thermal conductivity is also important for technical applications. Whereas high optical dispersion is an intrinsic property of all diamonds, their other properties vary depending on how the diamond was created.[53]
Crystallinity
Diamond can be one single, continuous crystal or it can be made up of many smaller crystals (polycrystal). Large, clear and transparent single-crystal diamonds are typically used in gemstones. Polycrystalline diamond consists of numerous small grains, which are easily seen by the naked eye through strong light absorption and scattering; it is unsuitable for gems and is used for industrial applications such as mining and cutting tools. Polycrystalline diamond is often described by the average size (or grain size) of the crystals that make it up. Grain sizes range from nanometers to hundreds of micrometers, usually referred to as "nanocrystalline" and "microcrystalline" diamond, respectively.[54]
Hardness
Synthetic diamond is the hardest material known,[55] where hardness is defined as resistance to scratching and is graded between 1 (softest) and 10 (hardest) using the Mohs scale of mineral hardness. Diamond has a hardness of 10 (hardest) on this scale.[56] The hardness of synthetic diamond depends on its purity, crystalline perfection and orientation: hardness is higher for flawless, pure crystals oriented to the [111] direction (along the longest diagonal of the cubic diamond lattice).[57] Nanocrystalline diamond produced through CVD diamond growth can have a hardness ranging from 30% to 75% of that of single crystal diamond, and the hardness can be controlled for specific applications. Some synthetic single-crystal diamonds and HPHT nanocrystalline diamonds (see hyperdiamond) are harder than any known natural diamond.[55][58][59]
Impurities and inclusions
Every diamond contains atoms other than carbon in concentrations detectable by analytical techniques. Those atoms can aggregate into macroscopic phases called inclusions. Impurities are generally avoided, but can be introduced intentionally as a way to control certain properties of the diamond. For instance, pure diamond is an electrical insulator, but diamond with boron added is an electrical conductor (and, in some cases, a superconductor),[60] allowing it to be used in electronic applications. Nitrogen impurities hinder movement of lattice dislocations (defects within the crystal structure) and put the lattice under compressive stress, thereby increasing hardness and toughness.[61]
Thermal conductivity
Unlike most electrical insulators, pure diamond is a good conductor of heat because of the strong covalent bonding within the crystal. The thermal conductivity of pure diamond is the highest of any known solid. Single crystals of synthetic diamond enriched in 12
C
(99.9%), isotopically pure diamond, have the highest thermal conductivity of any material, 30 W/cm·K at room temperature, 7.5 times higher than copper. Natural diamond's conductivity is reduced by 1.1% by the 13
C
naturally present, which acts as an inhomogeneity in the lattice.[62]
Diamond's thermal conductivity is made use of by jewelers and gemologists who may employ an electronic thermal probe to separate diamonds from their imitations. These probes consist of a pair of battery-powered thermistors mounted in a fine copper tip. One thermistor functions as a heating device while the other measures the temperature of the copper tip: if the stone being tested is a diamond, it will conduct the tip's thermal energy rapidly enough to produce a measurable temperature drop. This test takes about 2–3 seconds.[63]
Applications
Machining and cutting tools

Most industrial applications of synthetic diamond have long been associated with their hardness; this property makes diamond the ideal material for machine tools and cutting tools. As the hardest known naturally occurring material, diamond can be used to polish, cut, or wear away any material, including other diamonds. Common industrial applications of this ability include diamond-tipped drill bits and saws, and the use of diamond powder as an abrasive.[64] These are by far the largest industrial applications of synthetic diamond. While natural diamond is also used for these purposes, synthetic HPHT diamond is more popular, mostly because of better reproducibility of its mechanical properties. Diamond is not suitable for machining ferrous alloys at high speeds, as carbon is soluble in iron at the high temperatures created by high-speed machining, leading to greatly increased wear on diamond tools compared to alternatives.[65]
The usual form of diamond in cutting tools is micrometer-sized grains dispersed in a metal matrix (usually cobalt) sintered onto the tool. This is typically referred to in industry as polycrystalline diamond (PCD). PCD-tipped tools can be found in mining and cutting applications. For the past fifteen years, work has been done to coat metallic tools with CVD diamond, and though the work still shows promise it has not significantly replaced traditional PCD tools.[66]
Thermal conductor
Most materials with high thermal conductivity are also electrically conductive, such as metals. In contrast, pure synthetic diamond has high thermal conductivity, but negligible electrical conductivity. This combination is invaluable for electronics where diamond is used as a heat sink for high-power laser diodes, laser arrays and high-power transistors. Efficient heat dissipation prolongs the lifetime of those devices, and their high cost justifies the use of efficient, though relatively expensive, diamond heat sinks.[67] In semiconductor technology, synthetic diamond heat spreaders prevent silicon and other semiconducting materials from overheating.[68]
Optical material
Diamond is hard, chemically inert, and has high thermal conductivity and a low coefficient of thermal expansion. These properties make diamond superior to any other existing window material used for transmitting infrared and microwave radiation. Therefore, synthetic diamond is starting to replace zinc selenide as the output window of high-power CO2 lasers[69] and gyrotrons. Those synthetic diamond windows are shaped as disks of large diameters (about 10 cm for gyrotrons) and small thicknesses (to reduce absorption) and can only be produced with the CVD technique.[70][71]
Recent advances in the HPHT and CVD synthesis techniques improved the purity and crystallographic structure perfection of single-crystalline diamond enough to replace silicon as a diffraction grating and window material in high-power radiation sources, such as synchrotrons.[72][73] Both the CVD and HPHT processes are also used to create designer optically transparent diamond anvils as a tool for measuring electric and magnetic properties of materials at ultra high pressures using a diamond anvil cell.[74]
Electronics
Synthetic diamond has potential uses as a semiconductor,[75] because it can be doped with impurities like boron and phosphorus. Since these elements contain one more or one less valence electron than carbon, they turn synthetic diamond into p-type or n-type semiconductor. Making a p–n junction by sequential doping of synthetic diamond with boron and phosphorus produces light-emitting diodes (LEDs) producing UV light of 235 nm.[76] Another useful property of synthetic diamond for electronics is high carrier mobility, which reaches 4500 cm2/(V·s) for electrons in single-crystal CVD diamond.[77] High mobility is favorable for high-frequency field-effect transistors. The wide band gap of diamond (5.5 eV) gives it excellent dielectric properties. Combined with the high mechanical stability of diamond, those properties are being used in prototype high-power switches for power stations.[78]
Synthetic diamond transistors have been produced in the laboratory. They are functional at much higher temperatures than silicon devices, and are resistant to chemical and radiation damage. While no diamond transistors have yet been successfully integrated into commercial electronics, they are promising for use in exceptionally high power situations and hostile non-oxidizing environments.[79][80]
Synthetic diamond is already used as radiation detection device. It is radiation hard and has a wide bandgap of 5.5 eV (at room temperature). Diamond is also distinguished from most other semiconductors by the lack of a stable native oxide. This makes it difficult to fabricate surface MOS devices but does create the potential for UV radiation to get to the active semiconductor without absorption in a surface layer. Because of these properties, it is employed in applications such as the BaBar detector at the Stanford Linear Accelerator[81] and BOLD (Blind to the Optical Light Detectors for VUV solar observations).[82][83] A diamond VUV detector recently was used in the European LYRA program.
Conductive CVD diamond is a useful electrode under many circumstances.[84] Photochemical methods have been developed for covalently linking DNA to the surface of polycrystalline diamond films produced through CVD. Such DNA modified films can be used for detecting various biomolecules, which would interact with DNA thereby changing electrical conductivity of the diamond film.[85] In addition, diamonds can be used to detect redox reactions that cannot ordinarily be studied and in some cases degrade redox-reactive organic contaminants in water supplies. Because diamond is mechanically and chemically stable, it can be used as an electrode under conditions that would destroy traditional materials. As an electrode, synthetic diamond can be used in waste water treatment of organic effluents[86] and the production of strong oxidants.[87]
Gemstones

Synthetic diamonds for use as gemstones are grown by HPHT[32] or CVD[88] methods. They are available in yellow and blue, and to a lesser extent colorless (or white). The yellow color comes from nitrogen impurities in the manufacturing process while the blue color comes from boron.[30] Other colors such as pink or green are achievable after synthesis using irradiation.[89] Several companies also offer memorial diamonds grown using cremated remains.[90]
Gem-quality diamonds grown in a lab can be chemically, physically and optically identical (and sometimes superior) to naturally occurring ones. The mined diamond industry has undertaken legal, marketing and distribution countermeasures to protect its market from the emerging presence of synthetic diamonds. Man-made diamonds can be distinguished by spectroscopy in the infrared, ultraviolet, or X-ray wavelengths. The DiamondView tester from De Beers uses UV fluorescence to detect trace impurities of nitrogen, nickel or other metals in HPHT or CVD diamonds.[91]
At least one maker of laboratory-grown diamonds has made public statements about being "committed to disclosure" of the nature of its diamonds, and laser-inscribes serial numbers on all of its gemstones.[88] The company web site shows an example of the lettering of one of its laser inscriptions, which includes both the words "Gemesis created" and the serial number prefix "LG" (laboratory grown).[92]
See also
- Diamond simulant
- Diamond enhancement
- Isotopically pure diamond
- List of synthetic diamond manufacturers
- Material properties of diamond
- Memorial diamond
- Moissanite
- Poly(hydridocarbyne)
- J Willard Hershey
References
- ^ a b Din engleză: high-pressure high-temperature production method (metoda cu presiune și temperatură mare) și chemical vapor deposition production method (metoda de depunere chimică a vaporilor)
- ^ 16 C.F.R. Part 23: Guides For The Jewelry, Precious Metals, and Pewter Industries: Federal Trade Commission Letter Declining To Amend The Guides With Respect To Use Of The Term "Cultured", U.S. Federal Trade Commission, July 21, 2008.
- ^ Hannay, J. B. (). „On the Artificial Formation of the Diamond”. Proc. R. Soc. Lond. 30 (200–205): 450–461. doi:10.1098/rspl.1879.0144. JSTOR 113601.
- ^ C. Royère (). „Cuptorul electric al lui Henri Moissan la o sută de ani: legătură cu cuptorul electric, cuptorul solar, cuptorul plasmatic?”. Annales pharmaceutiques françaises. 57 (2): 116. PMID 10365467. Mai multe valori specificate pentru
|pages=
și|page=
(ajutor) - ^ Moissan, H. (). „Nouvelles expériences sur la reproduction du diamant”. Comptes Rendus. 118: 320.
- ^ Crookes, William (). Diamonds. London and New York's Harper Brothers. pp. 140 and up.
- ^ Ruff, O. (). „Über die Bildung von Diamanten”. Zeitschrift für anorganische und allgemeine Chemie. 99 (1): 73–104. doi:10.1002/zaac.19170990109.
- ^ Nassau, K. (). Gems made by Man. Chilton Book Co. pp. 12–25. ISBN 0-8019-6773-2.
- ^ Hershey, J. Willard (). The Book of Diamonds: Their Curious Lore, Properties, Tests and Synthetic Manufacture. Kessinger Publishing. pp. 123–130. ISBN 1-4179-7715-9.
- ^ Hershey, J. Willard (). Book of Diamonds. Heathside Press, New York. pp. 127–132. ISBN 0-486-41816-2.
- ^ „Permanent collection”. McPherson museum. Arhivat din original la . Accesat în .
- ^ a b Lonsdale, K. (). „Further Comments on Attempts by H. Moissan, J. B. Hannay and Sir Charles Parsons to Make Diamonds in the Laboratory”. Nature. 196 (4850): 104. Bibcode:1962Natur.196..104L. doi:10.1038/196104a0.
- ^ O'Donoghue, p. 473
- ^ Feigelson, R. S. (). 50 years progress in crystal growth: a reprint collection. Elsevier. p. 194. ISBN 0-444-51650-6.
- ^ Barnard, pp. 6–7
- ^ Parson, C. A. (). „Some notes on carbon at high temperatures and pressures”. Proceedings of the Royal Society of London. 79a (533): 532. Bibcode:1907RSPSA..79..532P. doi:10.1098/rspa.1907.0062. JSTOR 92683.
- ^ C.H. Desch (). „The Problem of Artificial Production of Diamonds”. Nature. 121 (3055): 799. Bibcode:1928Natur.121..799C. doi:10.1038/121799a0.
- ^ a b Hazen, R. M. (). The diamond makers. Cambridge University Press. pp. 100–113. ISBN 0-521-65474-2.
- ^ O'Donoghue, p. 474
- ^ a b Bovenkerk, H. P.; Bundy, F. P.; Chrenko, R. M.; Codella, P. J.; Strong, H. M.; Wentorf, R. H. (). „Errors in diamond synthesis”. Nature. 365 (6441): 19. Bibcode:1993Natur.365...19B. doi:10.1038/365019a0.
- ^ Hall, H. T. (). „Ultra-high pressure apparatus” (PDF). Rev. Sci. Instr. 31 (2): 125. Bibcode:1960RScI...31..125H. doi:10.1063/1.1716907.
- ^ Bundy, F. P.; Hall, H. T.; Strong, H. M. and Wentorf, R. H. (). „Man-made diamonds” (PDF). Nature. 176 (4471): 51. Bibcode:1955Natur.176...51B. doi:10.1038/176051a0.
- ^ a b Bovenkerk, H. P.; Bundy, F. P.; Hall, H. T.; Strong, H. M. and Wentorf, R. H. (). „Preparation of diamond” (PDF). Nature. 184 (4693): 1094. Bibcode:1959Natur.184.1094B. doi:10.1038/1841094a0.
- ^ Barnard, pp. 40–43
- ^ „ACS Award for Creative Invention”. American Chemical Society. Accesat în .
- ^ Liander, H. and Lundblad, E. (). „Artificial diamonds”. ASEA Journal. 28: 97.
- ^ Barnard, pp. 31–33
- ^ General Electric v. Sung, 843 F. Supp. 776: "granting production injunction against Iljin Diamond" cited in Epstein, M. A. (). Epstein on intellectual property. Aspen Publishers Online. p. 121. ISBN 0-7355-0319-2.
- ^ Hannas, W. C. (). The writing on the wall. University of Pennsylvania Press. pp. 76–77. ISBN 0-8122-3711-0.
- ^ a b R. C. Burns, V. Cvetkovic and C. N. Dodge (). „Growth-sector dependence of optical features in large synthetic diamonds”. Journal of Crystal Growth. 104 (2): 257. Bibcode:1990JCrGr.104..257B. doi:10.1016/0022-0248(90)90126-6.
- ^ Barnard, p. 166
- ^ a b Abbaschian, Reza; Zhu, Henry; Clarke, Carter (). „High pressure-high temperature growth of diamond crystals using split sphere apparatus”. Diam. Rel. Mater. 14 (11–12): 1916. Bibcode:2005DRM....14.1916A. doi:10.1016/j.diamond.2005.09.007.
- ^ Eversole, W. G. "Synthesis of diamond" U.S. Patent 3030188, April 17, 1962
- ^ Angus, John C. (). „Growth of Diamond Seed Crystals by Vapor Deposition”. J. Appl. Phys. 39 (6): 2915. Bibcode:1968JAP....39.2915A. doi:10.1063/1.1656693.
- ^ Deryagin, B. V. and Fedoseev, D. V. (). „Epitaxial Synthesis of Diamond in the Metastable Region”. Rus. Chem. Rev. 39. 39 (9): 783. Bibcode:1970RuCRv..39..783D. doi:10.1070/RC1970v039n09ABEH002022.
- ^ Spear and Dismukes, pp. 265–266
- ^ a b c Werner, M; Locher, R (). „Growth and application of undoped and doped diamond films”. Rep. Prog. Phys. 61 (12): 1665. Bibcode:1998RPPh...61.1665W. doi:10.1088/0034-4885/61/12/002.
- ^ a b Osawa, E (). „Recent progress and perspectives in single-digit nanodiamond”. Diamond and Related Materials. 16 (12): 2018. Bibcode:2007DRM....16.2018O. doi:10.1016/j.diamond.2007.08.008.
- ^ a b Galimov, É. M.; Kudin, A. M.; Skorobogatskii, V. N.; Plotnichenko, V. G.; Bondarev, O. L.; Zarubin, B. G.; Strazdovskii, V. V.; Aronin, A. S.; Fisenko, A. V. (). „Experimental Corroboration of the Synthesis of Diamond in the Cavitation Process”. Doklady Physics. 49 (3): 150. Bibcode:2004DokPh..49..150G. doi:10.1134/1.1710678.
- ^ „HPHT synthesis”. International Diamond Laboratories. Accesat în .
- ^ Barnard, p. 150
- ^ a b Ito, E. (). G. Schubert, ed. Multianvil cells and high-pressure experimental methods, in Treatise of Geophysics. 2. Elsevier, Amsterdam. pp. 197–230. ISBN 0-8129-2275-1.
- ^ Hall, H. T. (). „Ultrahigh-Pressure Research: At ultrahigh pressures new and sometimes unexpected chemical and physical events occur” (PDF). Science. 128 (3322): 445. Bibcode:1958Sci...128..445H. doi:10.1126/science.128.3322.445. PMID 17834381. Mai multe valori specificate pentru
|pages=
și|page=
(ajutor) - ^ Loshak, M. G. and Alexandrova, L. I. (). „Rise in the efficiency of the use of cemented carbides as a matrix of diamond-containing studs of rock destruction tool”. Int. J. Refractory Metals and Hard Materials. 19: 5. doi:10.1016/S0263-4368(00)00039-1.
- ^ Pal'Yanov, N.; Sokol, A.G.; Borzdov, M.; Khokhryakov, A.F. (). „Fluid-bearing alkaline carbonate melts as the medium for the formation of diamonds in the Earth's mantle: an experimental study”. Lithos. 60 (3–4): 145. Bibcode:2002Litho..60..145P. doi:10.1016/S0024-4937(01)00079-2.
- ^ a b Koizumi, S.; Nebel, C. E. and Nesladek, M. (). Physics and Applications of CVD Diamond. Wiley VCH. p. 50. ISBN 3-527-40801-0. Mai multe valori specificate pentru
|pages=
și|page=
(ajutor) - ^ Barjon, J.; Rzepka, E.; Jomard, F.; Laroche, J.-M.; Ballutaud, D.; Kociniewski, T.; Chevallier, J. (). „Silicon incorporation in CVD diamond layers”. Physica status solidi (a). 202 (11): 2177. Bibcode:2005PSSAR.202.2177B. doi:10.1002/pssa.200561920.
- ^ Kopf, R. F., ed. (). State-of-the-Art Program on Compound Semiconductors XXXIX and Nitride and Wide Bandgap Semiconductors for Sensors, Photonics and Electronics IV: proceedings of the Electrochemical Society. 2003–2011. The Electrochemical Society. p. 363. ISBN 1-56677-391-1.
- ^ Iakoubovskii, K.; Baidakova, M.V.; Wouters, B.H.; Stesmans, A.; Adriaenssens, G.J.; Vul', A.Ya.; Grobet, P.J. (). „Structure and defects of detonation synthesis nanodiamond”. Diamond and Related Materials. 9 (3–6): 861. Bibcode:2000DRM.....9..861I. doi:10.1016/S0925-9635(99)00354-4.
- ^ Decarli, P. and Jamieson, J. (). „Formation of Diamond by Explosive Shock”. Science. 133 (3467): 1821–1822. Bibcode:1961Sci...133.1821D. doi:10.1126/science.133.3467.1821. PMID 17818997.
- ^ Dolmatov, V. Yu. (). „Development of a rational technology for synthesis of high-quality detonation nanodiamonds”. Russian Journal of Applied Chemistry. 79 (12): 1913. doi:10.1134/S1070427206120019.
- ^ Khachatryan, A.Kh.; Aloyan, S.G.; May, P.W.; Sargsyan, R.; Khachatryan, V.A.; Baghdasaryan, V.S. (). „Graphite-to-diamond transformation induced by ultrasonic cavitation”. Diam. Relat. Mater. 17 (6): 931. Bibcode:2008DRM....17..931K. doi:10.1016/j.diamond.2008.01.112.
- ^ Spear and Dismukes, pp. 308–309
- ^ Zoski, Cynthia G. (). Handbook of Electrochemistry. Elsevier. p. 136. ISBN 0-444-51958-0.
- ^ a b Blank, V.; Popov, M.; Pivovarov, G.; Lvova, N.; Gogolinsky, K.; Reshetov, V. (). „Ultrahard and superhard phases of fullerite C60: comparison with diamond on hardness and wear” (PDF). Diamond and Related Materials. 7 (2–5): 427. Bibcode:1998DRM.....7..427B. doi:10.1016/S0925-9635(97)00232-X.
- ^ Read, P. G. (). Gemmology. Butterworth-Heinemann. pp. 49–50. ISBN 0-7506-6449-5.
- ^ Neves, A. J. and Nazaré, M. H. (). Properties, Growth and Applications of Diamond. IET. pp. 142–147. ISBN 0-85296-785-3.
- ^ Sumiya, H. (). „Super-hard diamond indenter prepared from high-purity synthetic diamond crystal”. Rev. Sci. Instrum. 76 (2): 026112. Bibcode:2005RScI...76b6112S. doi:10.1063/1.1850654.
- ^ Yan, Chih-Shiue; Mao, Ho-Kwang; Li, Wei; Qian, Jiang; Zhao, Yusheng; Hemley, Russell J. (). „Ultrahard diamond single crystals from chemical vapor deposition”. Phys. Stat. Solidi (a). 201 (4): R25. doi:10.1002/pssa.200409033.
- ^ Ekimov, E. A.; Sidorov, V. A.; Bauer, E. D.; Mel'Nik, N. N.; Curro, N. J.; Thompson, J. D.; Stishov, S. M. (). „Superconductivity in diamond” (PDF). Nature. 428 (6982): 542. arXiv:cond-mat/0404156
. Bibcode:2004Natur.428..542E. doi:10.1038/nature02449. PMID 15057827. Mai multe valori specificate pentru
|pages=
și|page=
(ajutor) - ^ Catledge, S. A.; Vohra, Yogesh K. (). „Effect of nitrogen addition on the microstructure and mechanical properties of diamond films grown using high-methane concentrations”. Journal of Applied Physics. 86: 698. Bibcode:1999JAP....86..698C. doi:10.1063/1.370787.
- ^ Wei, Lanhua; Kuo, P.; Thomas, R.; Anthony, T.; Banholzer, W. (). „Thermal conductivity of isotopically modified single crystal diamond”. Phys. Rev. Lett. 70 (24): 3764. Bibcode:1993PhRvL..70.3764W. doi:10.1103/PhysRevLett.70.3764. PMID 10053956. Mai multe valori specificate pentru
|pages=
și|page=
(ajutor) - ^ Wenckus, J. F. "Method and means of rapidly distinguishing a simulated diamond from natural diamond" U.S. Patent 4488821 December 18, 1984
- ^ Holtzapffel, C. (). Turning And Mechanical Manipulation. Holtzapffel. pp. 176–178. ISBN 1-879335-39-5.
- ^ Coelho, R.T.; Yamada, S.; Aspinwall, D.K.; Wise, M.L.H. (). „The application of polycrystalline diamond (PCD) tool materials when drilling and reaming aluminum-based alloys including MMC”. International journal of machine tools & manufacture. 35 (5): 761. doi:10.1016/0890-6955(95)93044-7.
- ^ Ahmed, W.; Sein, H.; Ali, N.; Gracio, J.; Woodwards, R. (). „Diamond films grown on cemented WC-Co dental burs using an improved CVD method”. Diamond and Related Materials. 12 (8): 1300. Bibcode:2003DRM....12.1300A. doi:10.1016/S0925-9635(03)00074-8.
- ^ M. Sakamoto, J. G. Endriz, D. R. Scifres (). „120 W CW output power from monolithic AlGaAs (800 nm) laser diode array mounted on diamond heatsink”. Electronics Letters. 28 (2): 197–199. doi:10.1049/el:19920123.
- ^ Ravi, Kramadhati V. et al. "Diamond-silicon hybrid integrated heat spreader" U.S. Patent 6924170, August 2, 2005
- ^ Harris, D. C. (). Materials for infrared windows and domes: properties and performance. SPIE Press. pp. 303–334. ISBN 0-8194-3482-5.
- ^ „The diamond window for a milli-wave zone high power electromagnetic wave output”. New Diamond. 15: 27. .
- ^ Nusinovich, G. S. (). Introduction to the physics of gyrotrons. JHU Press. p. 229. ISBN 0-8018-7921-3.
- ^ Khounsary, Ali M.; Smither, Robert K.; Davey, Steve; Purohit, Ankor (). „Diamond Monochromator for High Heat Flux Synchrotron X-ray Beams”. Proc. SPIE. 1739: 628–642. Bibcode:1993SPIE.1739..628K. doi:10.1117/12.140532. Arhivat din original la . Accesat în .
- ^ Heartwig, J.; et al. „Diamonds for Modern Synchrotron Radiation Sources”. European Synchrotron Radiation Facility. Accesat în .
- ^ Jackson, D. D.; Aracne-Ruddle, C.; Malba, V.; Weir, S. T.; Catledge, S. A.; Vohra, Y. K. (). „Magnetic susceptibility measurements at high pressure using designer diamond anvils”. Rev. Sci. Instrum. 74 (4): 2467. Bibcode:2003RScI...74.2467J. doi:10.1063/1.1544084.
- ^ Denisenko, A. and Kohn, E. (). „Diamond power devices. Concepts and limits”. Diamond and Related Materials. 14 (3–7): 491. Bibcode:2005DRM....14..491D. doi:10.1016/j.diamond.2004.12.043.
- ^ Koizumi, S.; Watanabe, K; Hasegawa, M; Kanda, H (). „Ultraviolet Emission from a Diamond pn Junction”. Science. 292 (5523): 1899. Bibcode:2001Sci...292.1899K. doi:10.1126/science.1060258. PMID 11397942. Mai multe valori specificate pentru
|pages=
și|page=
(ajutor) - ^ Isberg, J.; Hammersberg, J; Johansson, E; Wikström, T; Twitchen, DJ; Whitehead, AJ; Coe, SE; Scarsbrook, GA (). „High Carrier Mobility in Single-Crystal Plasma-Deposited Diamond”. Science. 297 (5587): 5587. Bibcode:2002Sci...297.1670I. doi:10.1126/science.1074374. PMID 12215638. Mai multe valori specificate pentru
|pages=
și|page=
(ajutor) - ^ Isberg, J.; Gabrysch, M.; Tajani, A. and Twitchen, D.J. (). „High-field Electrical Transport in Single Crystal CVD Diamond Diodes”. Advances in Science and Technology. 48: 73. doi:10.4028/www.scientific.net/AST.48.73.
- ^ Railkar, T. A.; Kang, W. P.; Windischmann, Henry; Malshe, A. P.; Naseem, H. A.; Davidson, J. L.; Brown, W. D. (). „A critical review of chemical vapor-deposited (CVD) diamond for electronic applications”. Critical Reviews in Solid State and Materials Sciences. 25 (3): 163. Bibcode:2000CRSSM..25..163R. doi:10.1080/10408430008951119.
- ^ "Designing diamond circuits for extreme environments", David Salisbury, Vanderbilt University Research News, Aug. 4, 2011
- ^ Bucciolini, M.; Borchi, E; Bruzzi, M; Casati, M; Cirrone, P; Cuttone, G; Deangelis, C; Lovik, I; Onori, S (). „Diamond dosimetry: Outcomes of the CANDIDO and CONRADINFN projects”. Nuclear Instruments and Methods A. 552: 189. Bibcode:2005NIMPA.552..189B. doi:10.1016/j.nima.2005.06.030.
- ^ „Blind to the Optical Light Detectors”. Royal Observatory of Belgium. Accesat în .
- ^ Benmoussa, A; Soltani, A; Haenen, K; Kroth, U; Mortet, V; Barkad, H A; Bolsee, D; Hermans, C; Richter, M (). „New developments on diamond photodetector for VUV Solar Observations”. Semiconductor Science and Technology. 23 (3): 035026. Bibcode:2008SeScT..23c5026B. doi:10.1088/0268-1242/23/3/035026.
- ^ Panizza, M. and Cerisola, G. (). „Application of diamond electrodes to electrochemical processes”. Electrochimica Acta. 51 (2): 191. doi:10.1016/j.electacta.2005.04.023.
- ^ Nebel, C.E.; Uetsuka, H.; Rezek, B.; Shin, D.; Tokuda, N.; Nakamura, T. (). „Inhomogeneous DNA bonding to polycrystalline CVD diamond”. Diamond and Related Materials. 16 (8): 1648. Bibcode:2007DRM....16.1648N. doi:10.1016/j.diamond.2007.02.015.
- ^ Gandini, D. (). „Oxidation of carbonylic acids at boron-doped diamond electrodes for wastewater treatment”. Journal of Applied Electrochemistry. 20 (12): 1345. doi:10.1023/A:1026526729357.
- ^ Michaud, P.-A. (). „Preparation of peroxodisulfuric acid using Boron-Doped Diamond thin film electrodes”. Electrochemical and Solid-State Letters. 3 (2): 77. doi:10.1149/1.1390963.
- ^ a b Yarnell, Amanda (). „The Many Facets of Man-Made Diamonds”. Chemical & Engineering News. American Chemical Society. 82 (5): 26–31. ISSN 0009-2347.
- ^ Walker, J. (). „Optical absorption and luminescence in diamond”. Rep. Prog. Phys. 42 (10): 1605. Bibcode:1979RPPh...42.1605W. doi:10.1088/0034-4885/42/10/001.
- ^ „Memorial Diamonds Deliver Eternal Life”. Reuters. . Accesat în .
- ^ O'Donoghue, p. 115
- ^ Laboratory Grown Diamond Report for Gemesis diamond, International Gemological Institute, 2007.
Bibliography
- A. S. Barnard (). The diamond formula: diamond synthesis-a gemological perspective. Butterworth-Heinemann. ISBN 0-7506-4244-0.
- Michael O'Donoghue (). Gems: their sources, descriptions and identification. Butterworth-Heinemann. ISBN 0-7506-5856-8.
- K. E. Spear and J. P. Dismukes (). Synthetic diamond. Wiley-IEEE. ISBN 0-471-53589-3.
- Christoph Wild "CVD Diamond Properties and useful Formula" CVD Diamond Booklet (2008) PDF
External links
- J. Davis (). „The New Diamond Age”. Wired Magazine (11.09). Accesat în .
- „Putting the Squeeze on Materials”. Accesat în .